Running concepts and foot strike patterns
- DanWatsonPhysio
- Apr 23, 2021
- 7 min read
Millions of people worldwide enjoy running as a way of improving fitness, reducing stress, promoting a feeling of wellbeing or simply for the act of running. Approximately 10% of the population regularly jog or run a few kilometres a day and more enthusiastic runners can run 5-10km and longer distances such as a marathon (42.2km) (1).
It has been hypothesised the need to cover large distances to gather food and endure long periods of hunting during human evolution has resulted in skeletal adaptations which by design promote efficient endurance running capabilities (2). However, despite this, running-related injuries are problematic affecting up to 75% of runners over their lifetime with injuries requiring days and months to recover (3). Commonly touted reasons for running-related injuries include the magnitude of impact forces, excessive foot pronation, asymmetrical loading and muscle function (4).
It can get very confusing and when these etiological factors are reviewed in isolation, one can build a case for each of their involvement in the development of running-related injuries. However, this can lead to an over complicated and convoluted approach to the rehabilitation of the injured runner and in my experience often results in delayed or unsatisfactory outcomes. During the remainder of this post, I discuss the role of the lower limb when we run and whether foot strike patterns may promote a normal or optimal running style.
By discussing this, I hope to provide a basic theoretical understanding of running accompanied by actionable steps to reduce injury risk in subsequent posts.
Fig,1
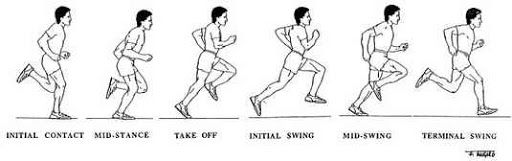
We transition from walking to running at approximately 2 metres per second (ms) or 7 kilometres per hour (kph) as it becomes more energy-efficient than walking at faster paces (5). This is due to the exploitation of tendons and ligaments of the lower limb, which are capable of storing and releasing energy much like a spring system. During running, we experience cyclical stance and swing phases. During stance, the foot makes contact with the ground absorbing and generating forces vertically, preventing us from collapsing and propelling us forward. During swing, the opposite leg moves through the air preparing for the stance phase contributing to horizontal momentum Fig,1.
The fit steady-state runner runs at approximately 3.2-4.2 ms (11.5-15.5 kph) with elite runners capable of a breathtaking 6 ms (21.5 kph) (6). To give perspective this means the fit steady-state runner completes a 10 kilometre run in 52-39 minutes whilst the elite runner would have completed it in a staggering 27-26 minutes.
Increasing running speeds are achieved through a combination of increasing our stride length and our stride frequency (7). At speeds between 2-7 ms increases in stride length are achieved through exerting increasingly larger support forces during the stance phase of running. To run beyond a speed of 7 ms which is commonly accepted as sprinting we transition to an even greater stride frequency by swinging the leg through the air in greater ranges and at a faster rate (8). To simplify this, running speeds under 7ms are foot and ankle dominant whilst speeds beyond 7ms the hip has an increasingly important role Fig2.
Fig 2.
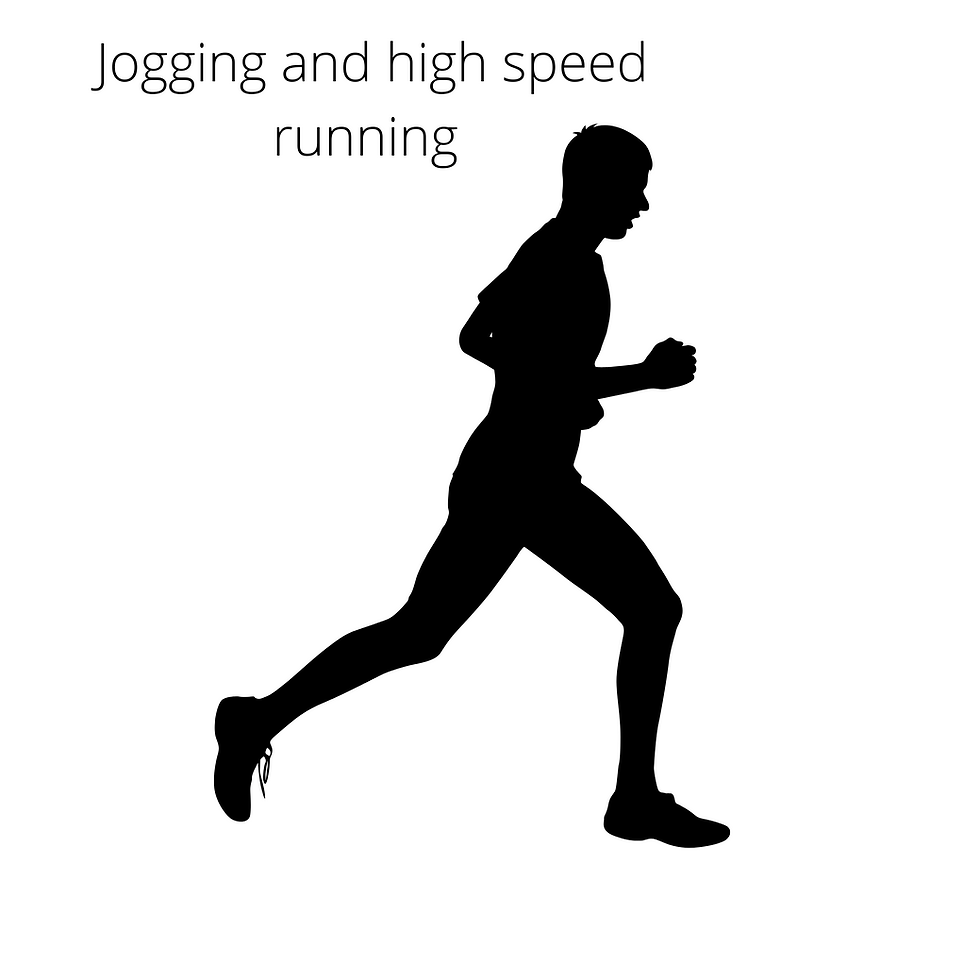
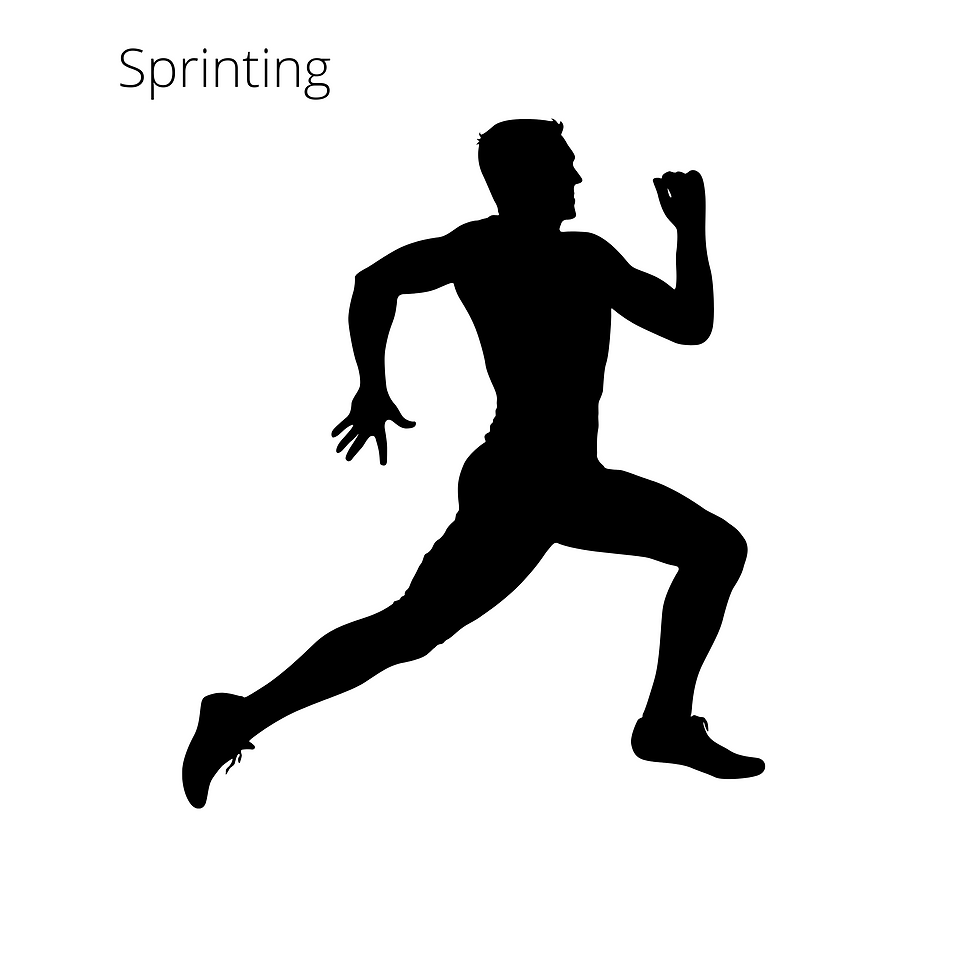
Steady-state runners are subject to up to 4 times bodyweight forces during the stance phase of running (9). These forces are transmitted from the ground, through the foot and ankle and up the stance leg into the spine and body. Understandably, this is widely considered the most injurious part of the running cycle. The ability to tolerate and utilise these forces is essential to reduce injury risk and optimise running efficiency.
Thanks to computer modelling and studying muscle activity patterns during running, we know that the calf complex, quadriceps and gluteal muscles are the main contributors to absorbing and generating these high forces during the stance phase of running. The calf complex and quadriceps muscles in particular accountant for approximately 75% of this total vertical support. Furthermore, the soleus, the largest muscle within the calf complex, is responsible for up to a monumental 50% of this vertical support (10). Contrary to popular belief, the primary role of the gluteal muscles is to provide pelvic and trunk support during stance and thus indirectly rather than directly contributes to propulsion (11).
The way in which muscles work and interact with tendons to absorb and generate forces is an important concept in the context of running efficiency. In order to grasp this concept, you can think of muscles as either energy generators or energy conservers during a specific task. The architecture of the individual muscles determines how well suited it is to performing these roles and it has been demonstrated these features are remarkably consistent among all individuals (11) so the principles are true for all runners.
The important soleus muscle for instance is an energy generator actively shortening throughout the stance phase of running. During the first half of stance, the soleus transfers energy to the Achilles tendon, which is stored and then utilised along with continued muscle shortening resulting in energy amplification and thus propulsion in the latter half of stance. In contrast, the vastus lateralis, the largest of the quadriceps does not actively shorten but rather works isometrically (statically) despite changes in the length of the tendon. This results in a spring-like exchange between the body, vastus lateralis and quadriceps tendon promoting energy conservation (12).
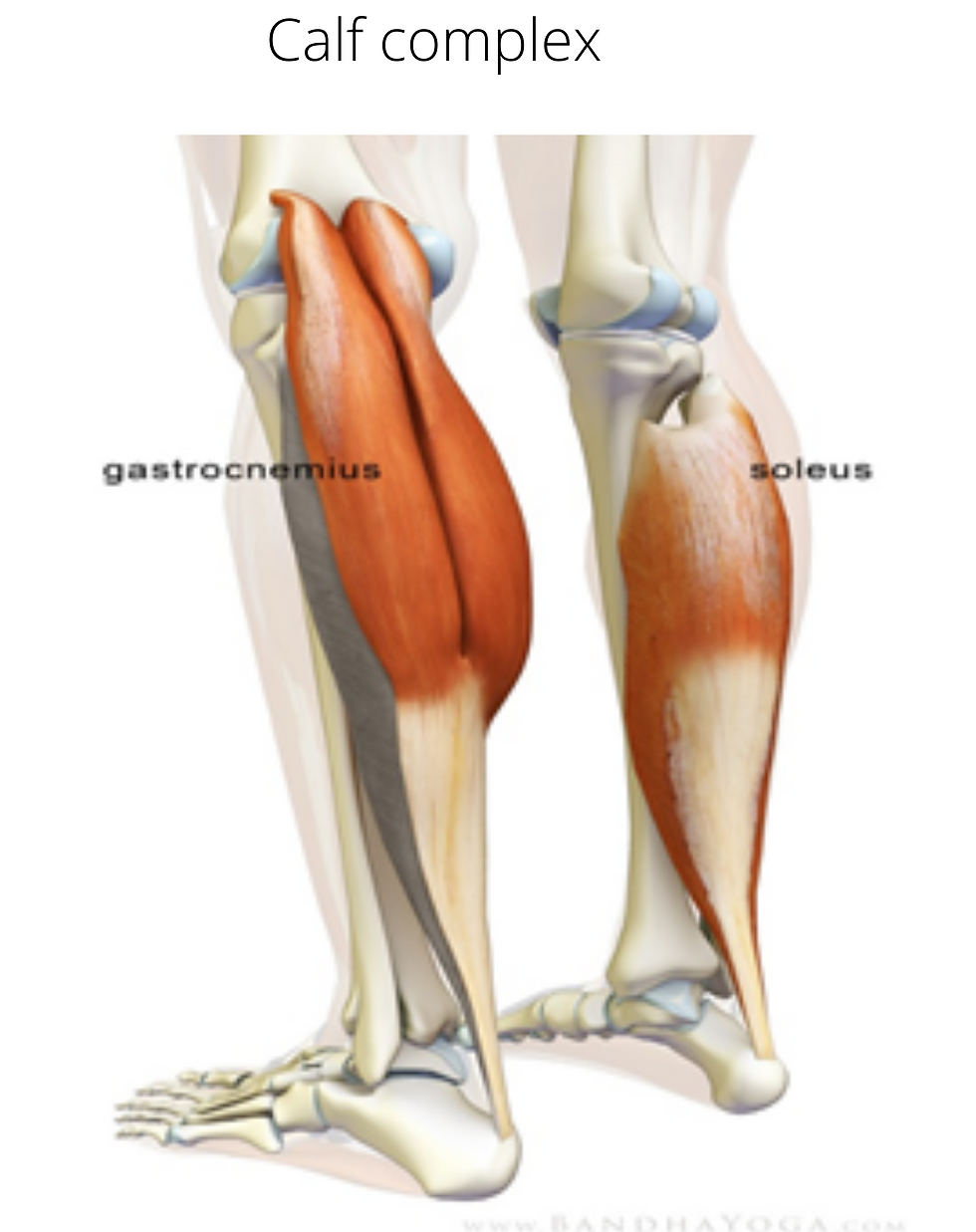
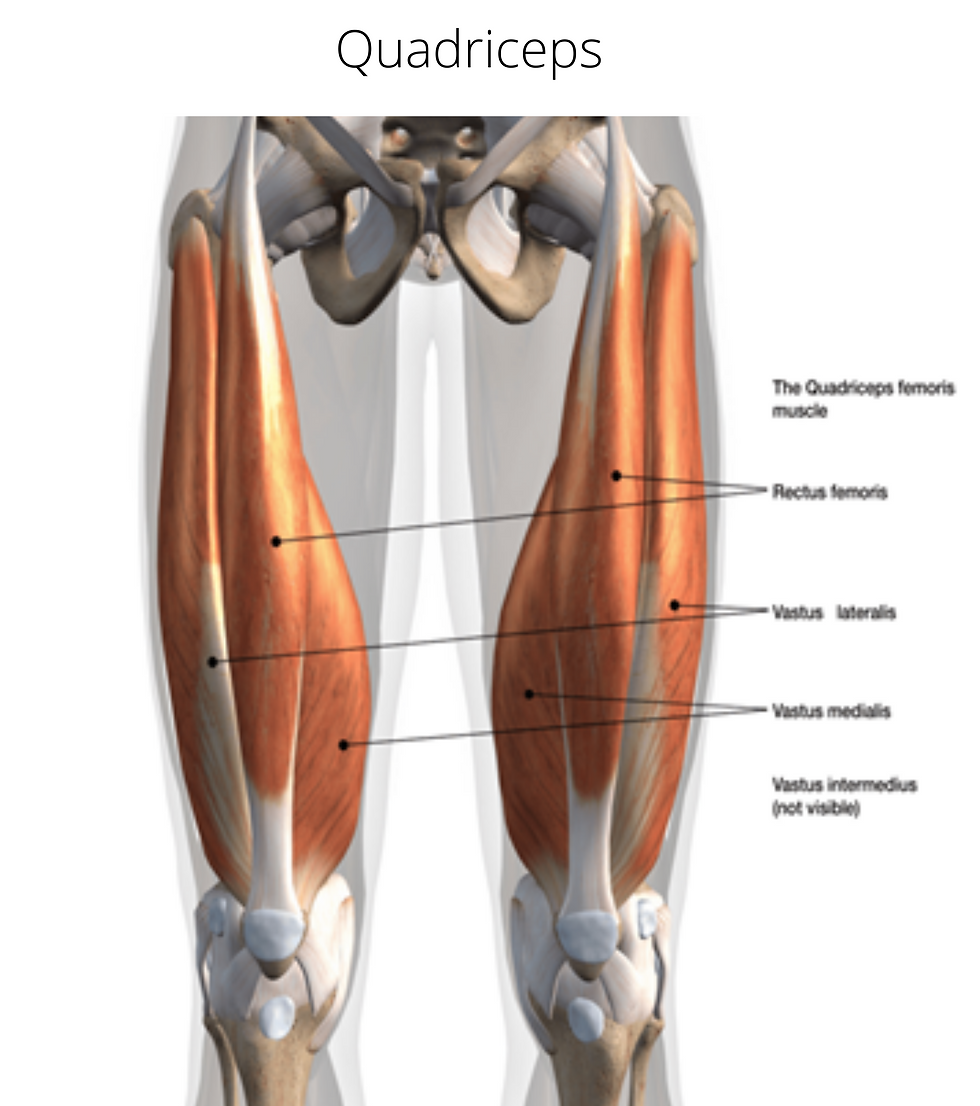
Therefore, it would seem logical to run in a way that maximises these inherent architectural features. This prompts the question is there an optimal running style and if so what does it look like and how can we achieve it? To answer this comprehensively is beyond the scope of this post but in the context of improving running efficiency and reducing injury risk, foot strike patterns have been a source of interesting debate.
Since the introduction of the modern running shoe in the 1970s, it is reported that 75-80% of runner’s rearfoot strike, whereby the heel strikes the ground on initial contact (13). Prior to the modern running shoe in which a thick cushioned sole provides some shock absorption (14) runners typically ran barefoot or with minimal support potentially adopting a mid or forefoot strike pattern which negated the need for external cushioning at the heel (15) Fig 3. These foot strike patterns result in different joint positions at the foot/ankle, knee and hip during the running cycle.
This is important, as joint position influences muscles ability to contract maximally and the speed at which it can produce the force. Furthermore, joint position influences passive stiffness of tendons and fascial tissues, which are important to support and amplify energy storage and release. In the important calf muscles for instance it has been shown maximal strength and contraction speed are greater in neutral ankle positions (16).
Habitual mid and forefoot strikers make initial contact with the ground in a more neutral ankle position and tend to have a greater degree of knee flexion during running compared to rearfoot strikers (17, 18) Fig 4. This is associated with up to 3 times less vertical ground reactions forces and crucially the rate at which the body is subjected to this load is up to 7 times less (19). Considering runners typically make 600+ contacts with the ground during a kilometre it would seem both more efficient and protective to reduce overall load exposure.
Fig3.
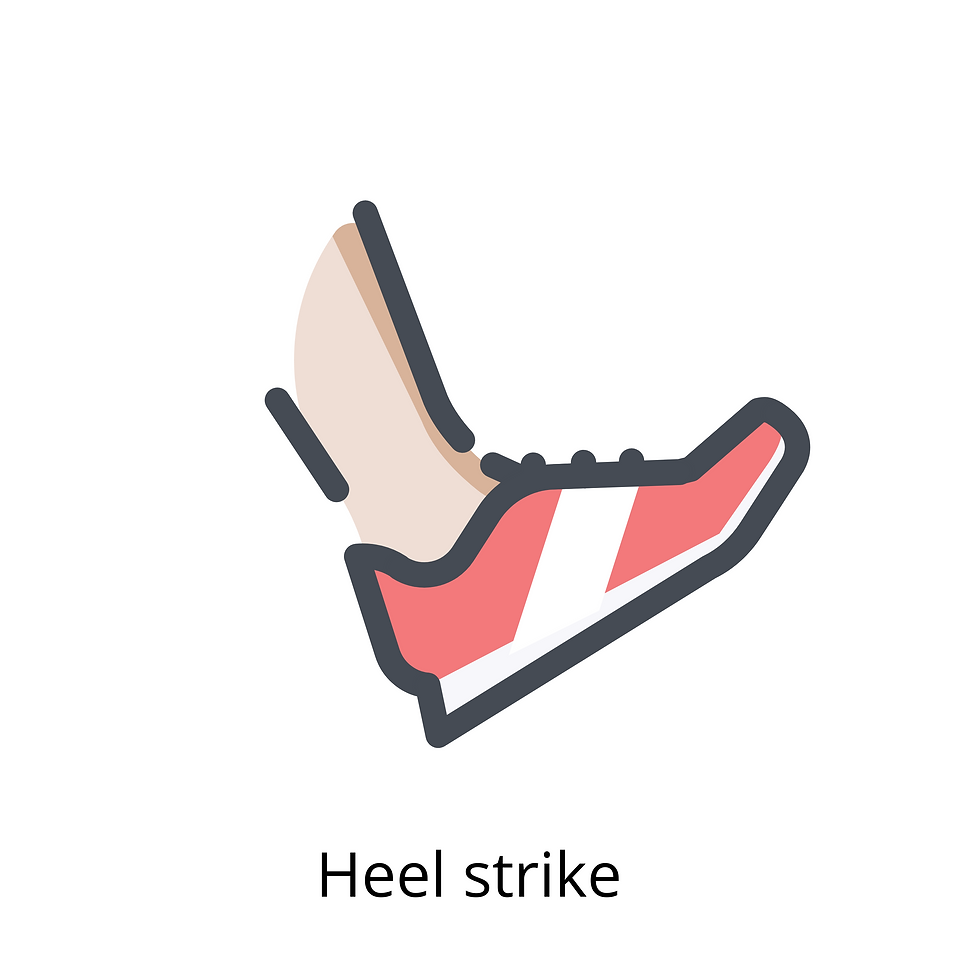
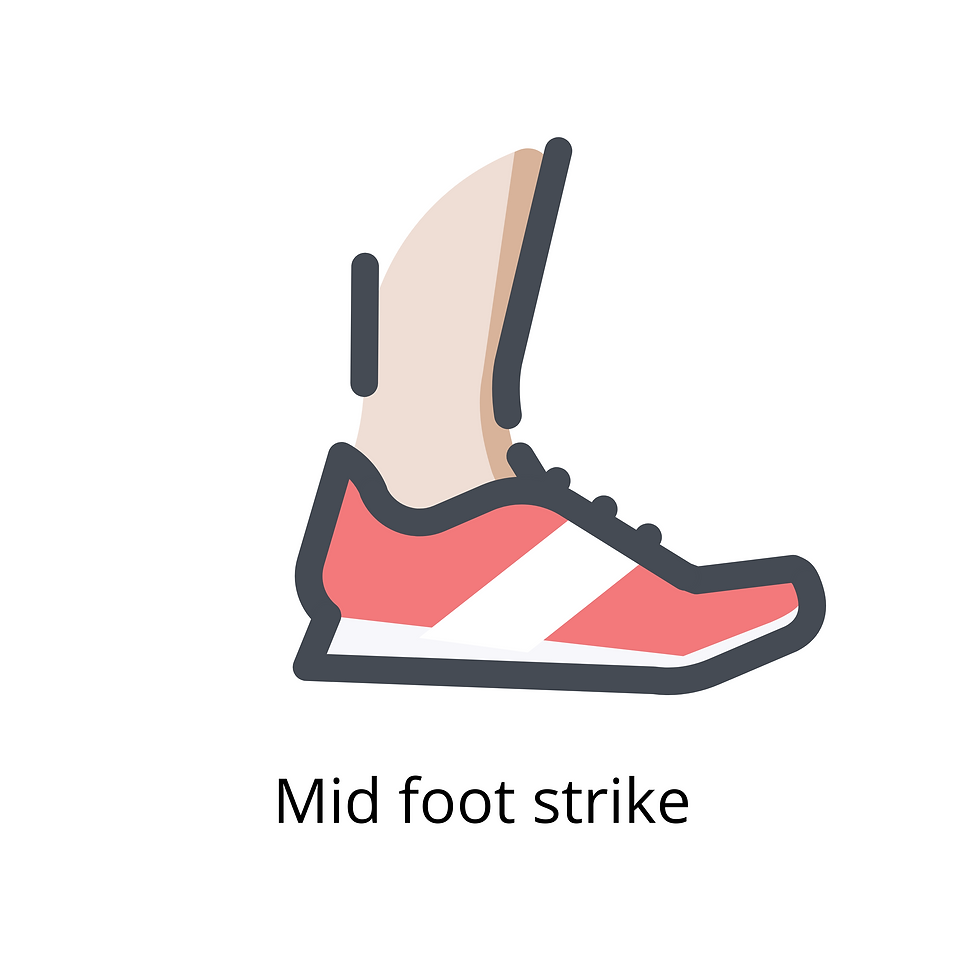
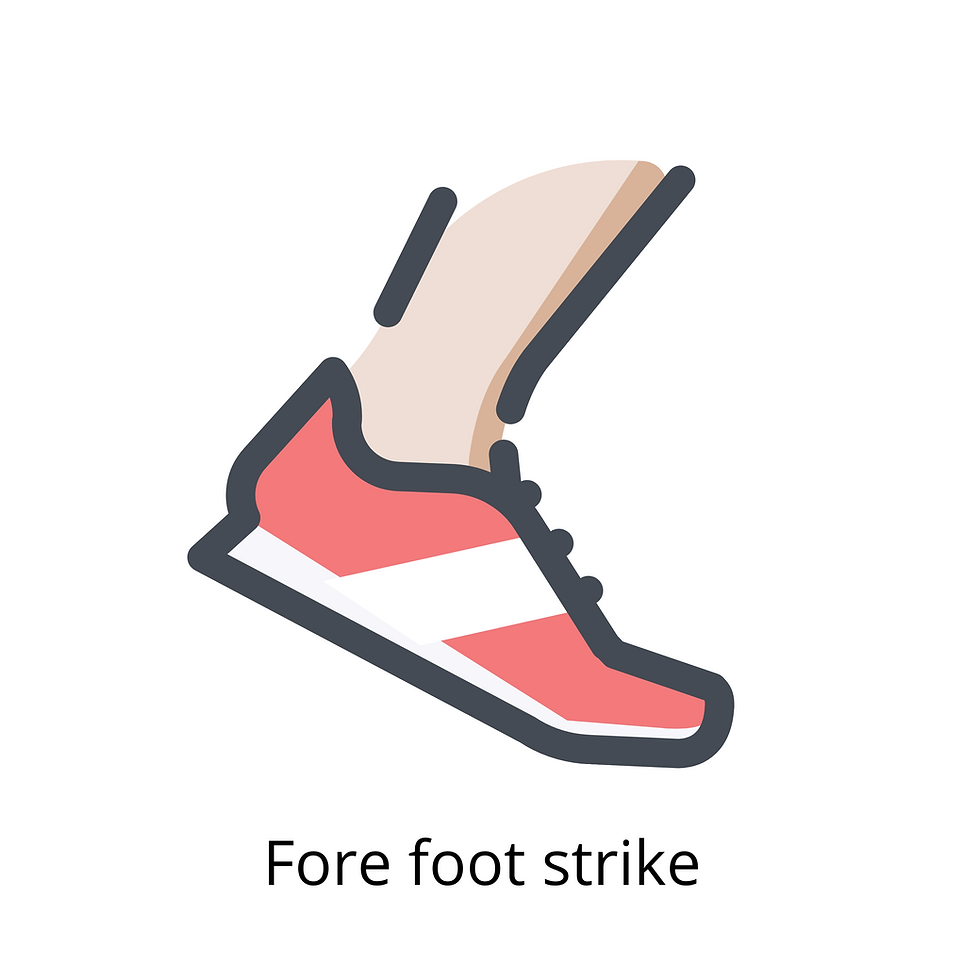
Paradoxically elite level marathon runners do not consistently demonstrate a mid or forefoot strike pattern (20) and mid or forefoot strikers do not consistently win races (21) as one may expect. This is a reflection of the complex variables involved in running and implies foot strike pattern is not an essential determinate of performance in elite distance runners. Sprinters on the other hand consistently forefoot strike, which is likely an adaptive response whereby the calf muscles shorten to continue producing high forces at great speeds in response to shorter ground contact times (22). This gives credence to the view foot strike selection may be task-specific.
Despite anecdotal evidence that mid and forefoot strike patterns reduce injury (22), there are no long-term prospective studies to confirm this. However, factors that are associated with lower limb injury mentioned at the start of this post can be modified when adopting a mid/fore foot-striking pattern. Intrinsic foot and calf muscle strength and size increased after a period of wearing minimally supportive footwear (23). Runners with high asymmetrical vertical loading rates significantly improved when adopting barefoot running (24). Further still, peak knee stress was reduced by 14% when rearfoot strikers converted to forefoot striking over a 12-week running retraining programme (25).
This would suggest there is a role for mid/forefoot striking in the injured runner and potentially as a method of reducing injury risk. As discussed earlier footwear may influence foot strike pattern but interestingly novel research has demonstrated that a simple instruction to run ‘lightly, softly and quietly’ results in runners adopting a mid/forefoot strike pattern regardless of footwear conditions (26).
Fig 4
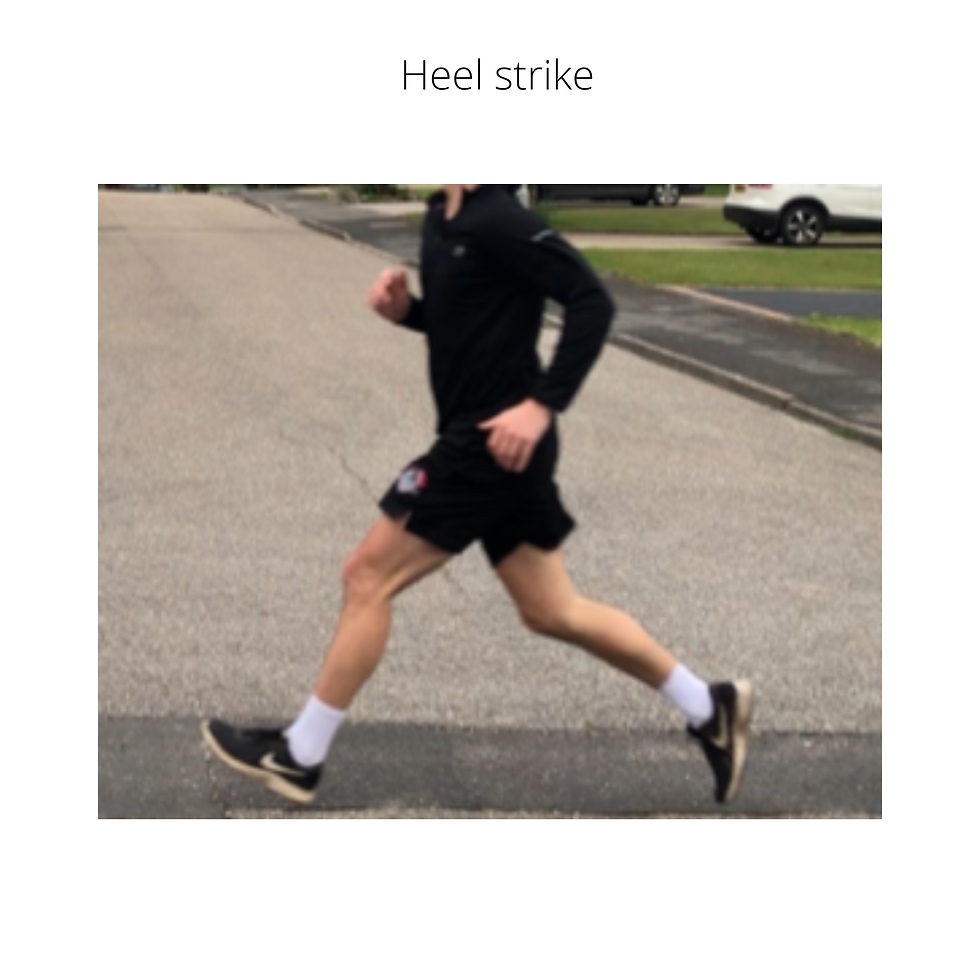
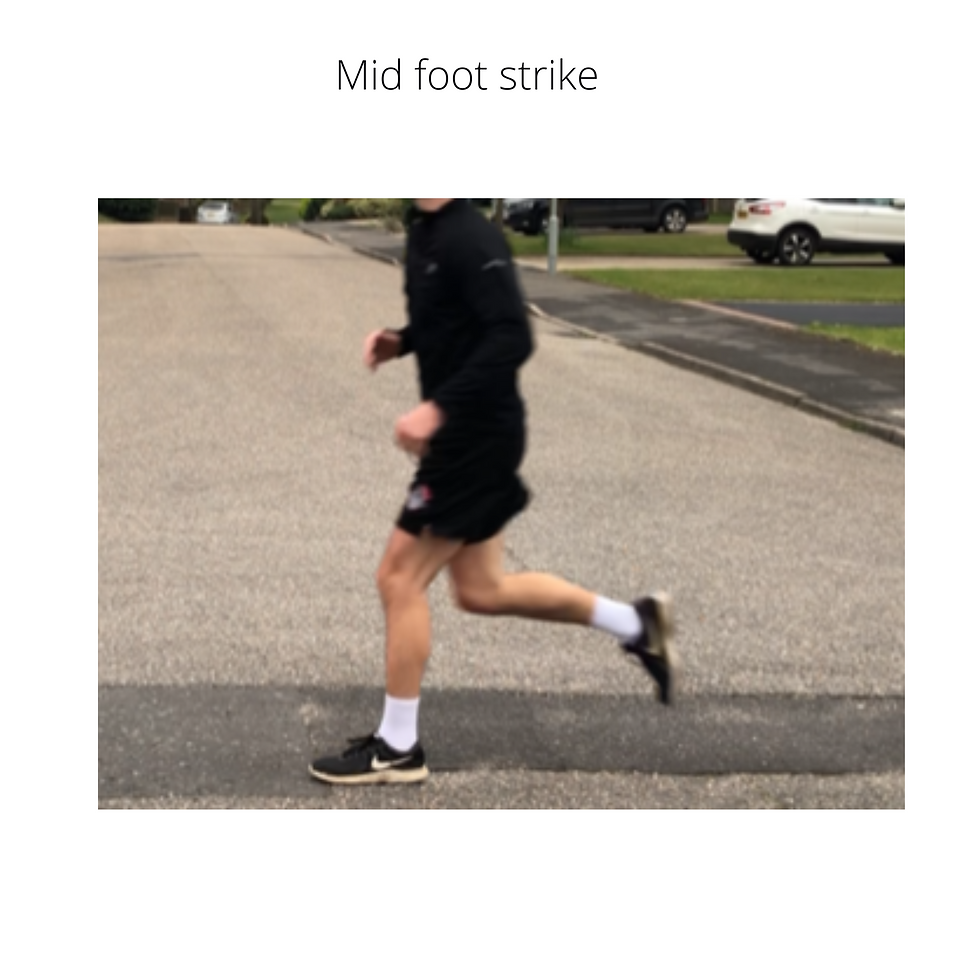
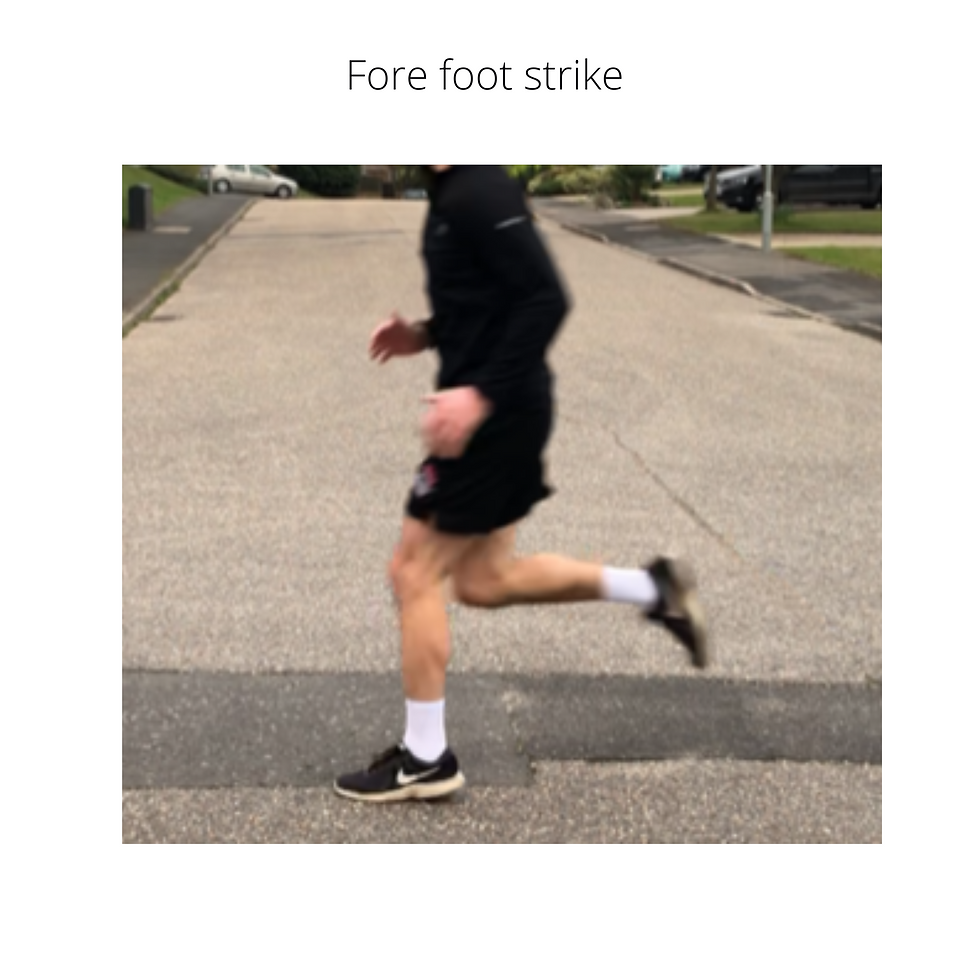
However, despite compelling evidence to support a transition from rear to mid and forefoot striking some authors have suggested this cannot be recommended to recreational runners (27). It is argued contradictory evidence exists that impact forces and loading rates are not as greatly reduced in mid and forefoot strikers as previously demonstrated. Furthermore, the effect of these changes is less apparent in novice runners regardless of foot strike, suggesting experienced runners may be more adaptable to reducing loading rates.
From a muscular perspective, the function of the soleus muscle may actually be optimised in a rearfoot striking position. Greater soleus forces are produced in a dorsiflexed ankle (which is associated with rearfoot striking) compared to a plantarflexed position typical of forefoot striking (28, 29). Given the important role the soleus plays in vertical support forces, this may be advantageous in the preparation of load absorption and generation.
It is also hypothesised that changing footstrike pattern will not reduce injury incidence but rather lead to stress in different locations due to changes in joint and muscle forces. Novice runners who typically rearfoot strike report more knee injuries and in contrast mid and forefoot strikers report increased foot and ankle injuries (30) Fig 5. So regardless of foot strike pattern, there is likely to be an injury risk.
Fig 5.
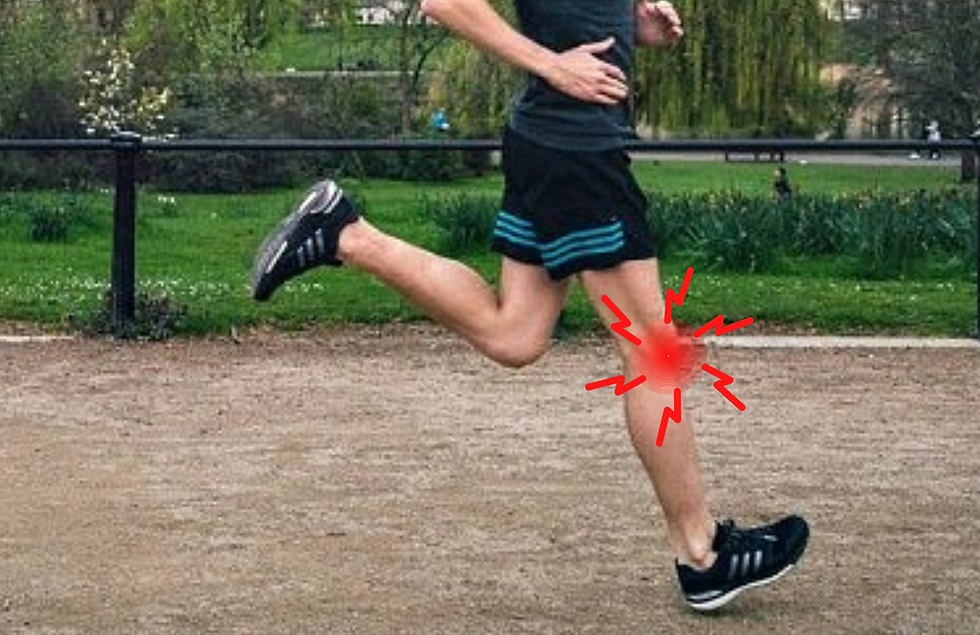
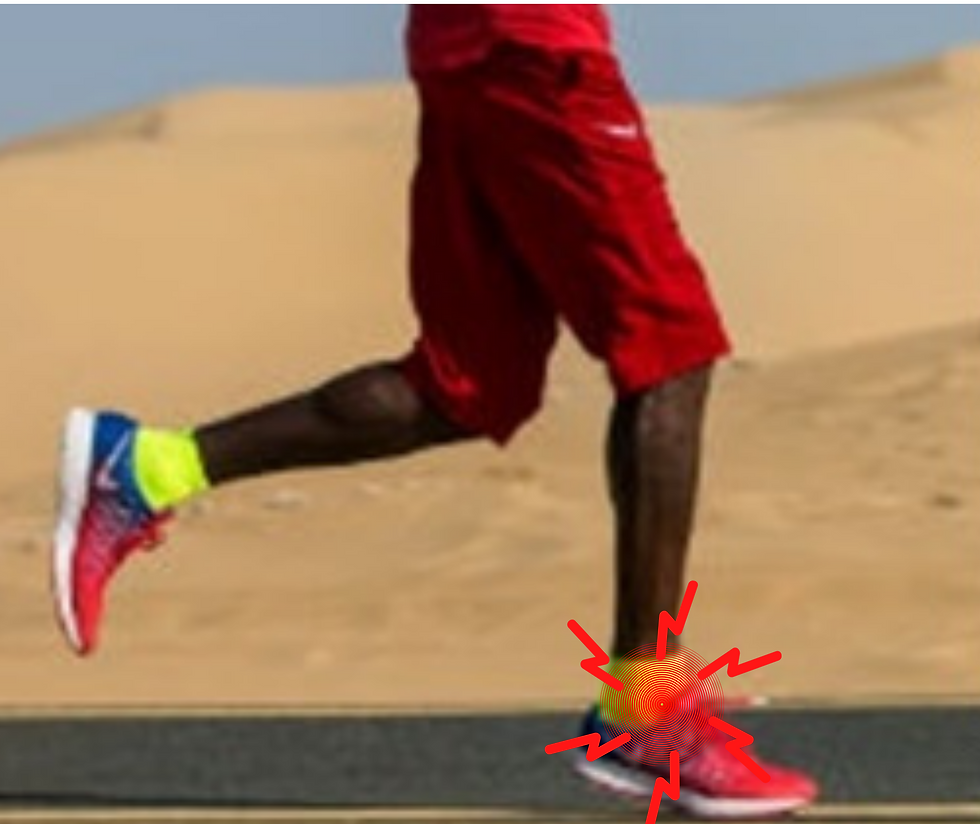
Adopting a new foot strike pattern also requires greater energy expenditure (31). Therefore, unless a dedicated training programme is followed the majority of runners will revert to their habitual foot strike pattern, especially under fatigue. So for runners whereby a change in foot strike pattern may be warranted this should be preceded by a period of physical preparation and running volume graduation.
In the context of running and foot strike patterns, there is unlikely to be a normal or optimal running style that all runners should adopt. However, by understanding the stresses that running places on the body and the important role and function of lower limb muscles and joints the recreational runner can prepare and recover adequately to reduce their injury risk. In subsequent posts, I shall give actionable advice to compliment the underlying mechanisms discussed today that can move the needle for those wishing to start and continue enjoying their running.
Thanks for reading
Comments